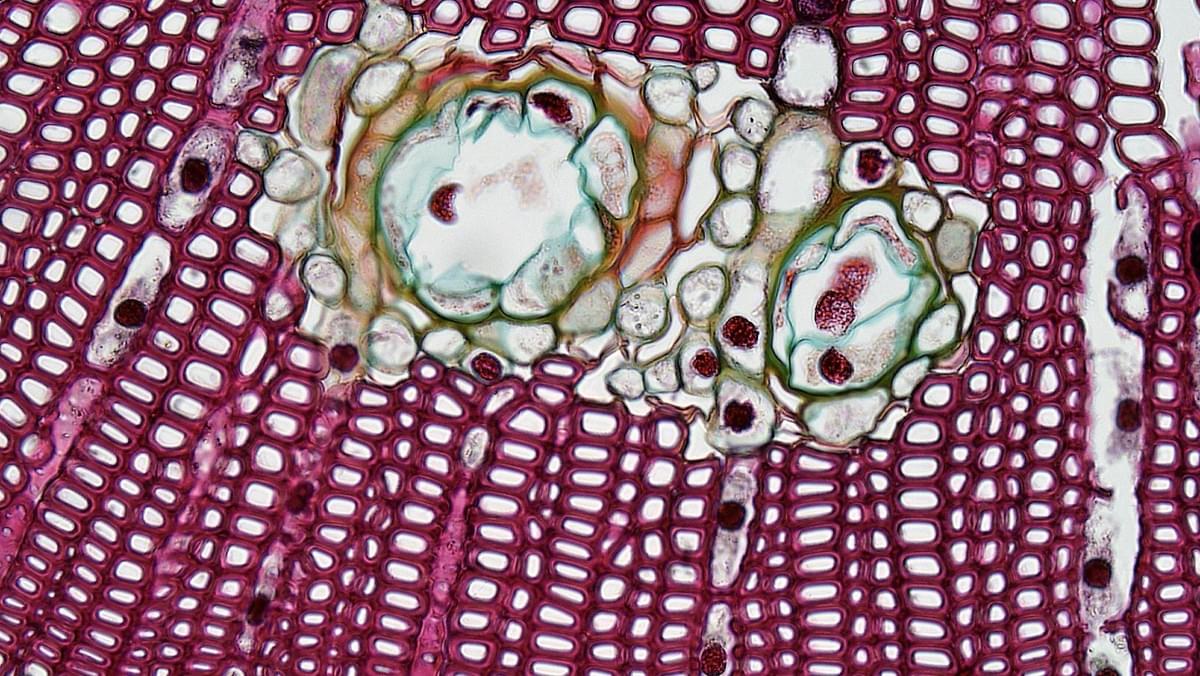
Small molecules are organic compounds that possess a low molecular weight and are thus able to pass through cell walls, engaging targets within the cell, as with drugs such as aspirin and ibuprofen. The cell has an internal composition defined by a plasma membrane barrier. A majority of biological molecules cannot diffuse through this phospholipid bilayer. It effectively prevents a free molecule exchange between the cell’s external and internal environments (cytoplasm).
The plasma membrane does provide selective permeability to some small molecules. There are several ways in which this is accomplished, including passive diffusion, facilitated diffusion, ion channels, and ATO hydrolysis-enabled active transport.
The simplest mechanism, passive diffusion, is a nonselective process in which the molecules dissolve into the phospholipid bilayer, diffuse across it, and finally dissolve into the aqueous solution on the inner side of the membrane. This process is determined by relative molecular concentrations outside and inside the cell. The net flow of molecules is from high-concentration compartments to low-concentration compartments.
Beyond their small size, the passive molecules must also be relatively hydrophobic to pass through the phospholipid bilayer. Thus, passive diffusion allows diffusion by gases such as O2 and CO2 and small uncharged polar molecules such as ethanol and H2O. Small hydrophobic molecules such as benzene can also pass through.
No charged molecules of any size may enter through passive diffusion, and larger uncharged polar molecules, such as glucose, are also barred. The cells must activate specific channels and transport proteins to shepherd most biological molecules in and out of the membrane.
Like its passive counterpart, facilitated diffusion is determined by the relative concentrations of molecules inside and outside the cells (with charged molecules, the electric potential across the membrane determines the direction of flow). However, the process has a major difference: transported molecules don’t simply dissolve in the phospholipid bilayer. Rather, cellular proteins mediate their passage and prevent direct interactions with the hydrophobic cell interior. This allows charged and polar molecules, including amino acids, carbs, ions, and nucleosides, to cross the plasma barrier.
Two basic types of proteins are involved in facilitated diffusion: carrier proteins bind to specific molecules and undergo conformational changes that enable them to pass through the membrane until they are released within the cell. A prime example of a carrier protein is the glucose transporter, which is present in red blood cells and regulates cells’ uptake of glucose, a primary metabolic energy source.
Unlike carrier proteins, channel proteins do not bind to and transport molecules; rather, they create open pores that allow any molecule of an appropriate charge and size to be freely diffused through the lipid bilayer. An example is the water channel proteins (aquaporins) that many cells possess. This makes the crossing of H2O molecules much faster than passive diffusion allows.
Ion channels are present in all cells and are particularly important in muscle and nerve cells. Their regulating opening and closing enables electric signals to be transmitted, muscles quickly utilized to generate movement, and sensations such as pain felt. Transport through these channels is extremely rapid, with a million ions per second flowing across open channels. This rate exceeds the flow rate of carrier proteins by a factor of more than a thousand.
Whether channel or carrier proteins are involved, facilitated diffusion has a net flow of molecules that is always energetically downhill and in a direction defined by electrochemical gradients within the membrane. In frequent situations, the cell is tasked with transporting molecules in a direction counter to concentration gradients. In such cases, a coupled reaction enables active transport, with molecules transported uphill in an energetically unfavorable direction.
ATP hydrolysis is the catabolic reaction that drives many such processes. Chemical energy stored within the high-energy phosphoanhydride bonds of adenosine triphosphate is broken down by water and released. This allows for regulated concentrations of elements, inside and outside the cell, that would not otherwise exist. For example, chlorine and sodium ions are around 10 times more concentrated in extracellular fluids (such as blood) than in the cytoplasm within the cell.